Overview of Laser Metal Deposition (LMD)
Laser Metal Deposition (LMD) is a groundbreaking additive manufacturing (AM) technology that uses a high-powered laser to melt and fuse metal powder or wire onto a substrate, layer by layer, to create three-dimensional structures. Whether you’re in the aerospace, automotive, or medical industry, LMD provides a robust solution for complex metal parts that require precision, durability, and efficiency.
Imagine needing to repair a worn-out turbine blade or create a custom implant. Traditional manufacturing methods might be too slow, too costly, or simply not precise enough. LMD swoops in like a superhero, using lasers to weld metal powders into the perfect shape, layer by meticulous layer. The result? Highly accurate and strong components that might even outshine the originals.
But how does it work? What are the materials used? And most importantly, how can it benefit your business? In this detailed guide, we’ll dive deep into LMD’s ins and outs, from the specific metal powders it uses to its pros and cons, and even a handy FAQ section to clear up any lingering questions.
So, buckle up as we explore the fascinating world of Laser Metal Deposition!
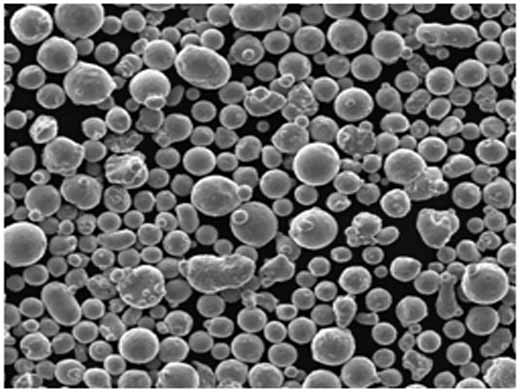
What is Laser Metal Deposition (LMD)?
LMD is a type of additive manufacturing, also known as 3D printing, where materials are added instead of removed. The process involves using a focused laser beam to melt metal powder or wire, which is then deposited onto a substrate. This technology allows for precise control over the material, enabling the creation of complex geometries and high-performance metal components.
How Does Laser Metal Deposition Work?
Laser Metal Deposition works by delivering metal powder through a nozzle into a laser beam, which melts the powder and fuses it to a base material (substrate). As the laser moves, it builds up layers of metal to form the desired shape. It’s like building a wall, but instead of bricks, you’re using tiny grains of metal, and instead of mortar, a high-energy laser binds everything together.
- Laser Source: The type and power of the laser determine how the metal powder will melt and solidify. Fiber lasers are commonly used for their efficiency and precision.
- Powder Feed System: Metal powders are fed into the laser beam via a nozzle. The consistency of the powder flow is crucial for achieving uniform layers.
- Motion Control: The motion of the laser and powder feed system is precisely controlled, often with CNC or robotic systems, to create the desired geometry.
This precision means that LMD is not just about building new parts. It can also repair existing ones by adding material where needed, which is particularly useful for expensive components in industries like aerospace.
Applications of Laser Metal Deposition (LMD)
Laser Metal Deposition is a versatile process with a wide range of applications across various industries. From repairing high-value components to creating complex, custom-designed parts, LMD is making waves in manufacturing.
Key Applications:
Industry | Application | Benefits |
---|---|---|
Aerospace | Turbine blade repair, engine components | Reduced downtime, extended life of parts |
Automotive | Gear and crankshaft repairs, custom parts | Improved performance, cost-effective production |
Medical | Custom implants, dental components | High precision, biocompatibility |
Tooling | Mold repair, die restoration | Longer tool life, reduced lead time |
Oil & Gas | Valve seats, drilling components | Resistance to harsh environments, reduced replacement cost |
Power Generation | Turbine repairs, component manufacturing | Improved efficiency, reduced maintenance costs |
Defense | Armament components, repair of critical equipment | High strength, durability in extreme conditions |
Energy | Wind turbine components, nuclear reactor parts | Sustainability, high performance in critical operations |
Construction | Custom structural components, heavy machinery repairs | Strength, flexibility, cost-effectiveness |
Marine | Propeller repairs, ship engine components | Corrosion resistance, longevity in marine environments |
Each of these industries has unique requirements, and LMD’s flexibility allows it to meet them all with ease. Whether it’s the high-strength materials needed for aerospace or the biocompatible metals used in medical implants, LMD can handle it.
Specific Metal Powders Used in LMD
The choice of metal powder is crucial in LMD, as it directly affects the quality, durability, and functionality of the final product. Here, we explore some of the specific metal powders commonly used in LMD, along with their compositions and characteristics.
Top 10 Metal Powders for LMD:
Metal Powder | Composition | Properties | Characteristics |
---|---|---|---|
Inconel 625 | Nickel-chromium-molybdenum-niobium alloy | High strength, excellent corrosion resistance | Used in aerospace, marine, chemical industries |
Titanium Ti-6Al-4V | Titanium-aluminum-vanadium alloy | High strength-to-weight ratio, biocompatibility | Ideal for medical implants, aerospace |
Stainless Steel 316L | Iron-chromium-nickel-molybdenum alloy | Corrosion resistance, good mechanical properties | Common in medical, food processing industries |
Cobalt-Chrome (Co-Cr) | Cobalt-chromium alloy | Wear resistance, biocompatibility | Used in dental, orthopedic implants |
Aluminum AlSi10Mg | Aluminum-silicon-magnesium alloy | Lightweight, good thermal properties | Popular in automotive, aerospace applications |
Hastelloy X | Nickel-chromium-iron-molybdenum alloy | Heat resistance, oxidation resistance | Suitable for high-temperature environments |
Maraging Steel 18Ni300 | Nickel-cobalt-molybdenum-titanium alloy | Ultra-high strength, good toughness | Used in tooling, aerospace |
Nickel 718 | Nickel-chromium-iron alloy | High tensile strength, creep resistance | Widely used in turbine engines, aerospace |
Tool Steel H13 | Chromium-molybdenum-vanadium alloy | High hardness, wear resistance | Ideal for tooling, die casting molds |
Copper-CrZr | Copper-chromium-zirconium alloy | Excellent thermal conductivity, strength | Used in heat exchangers, electrical components |
These metal powders are carefully chosen based on the specific requirements of the application, including factors such as strength, corrosion resistance, and biocompatibility.
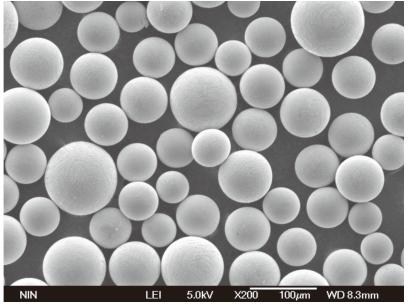
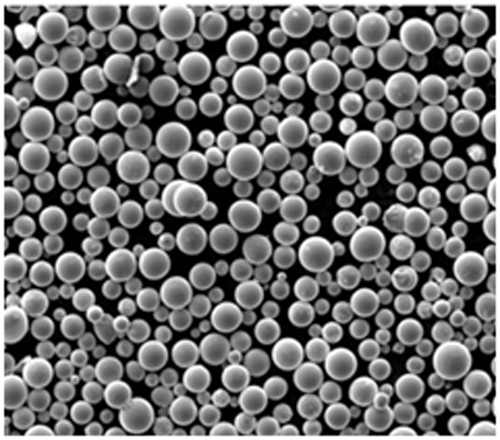
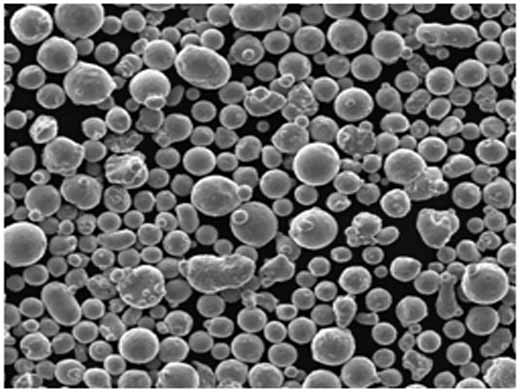
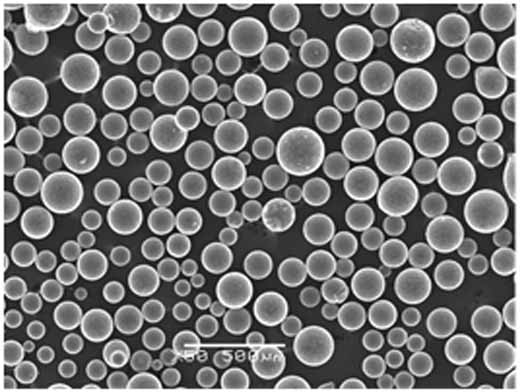
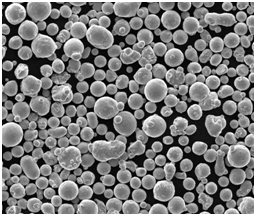
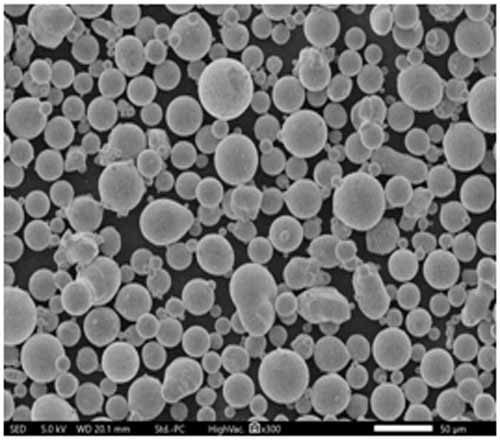
Properties and Characteristics of Laser Metal Deposition (LMD)
Understanding the properties and characteristics of LMD is essential to fully appreciate its capabilities and potential limitations. Let’s break down these aspects to get a clearer picture of what makes LMD tick.
Material Properties:
Property | Description |
---|---|
Density | LMD components typically have high density, comparable to forged parts. |
Microstructure | Fine microstructures can be achieved, leading to high strength and toughness. |
Surface Finish | The surface finish can vary depending on the powder and process parameters, often requiring post-processing. |
Porosity | Minimal porosity is achievable with optimized parameters, though this can vary based on the material and application. |
Residual Stresses | LMD can introduce residual stresses, which may need to be relieved through heat treatment. |
Process Characteristics:
Characteristic | Details |
---|---|
Layer Thickness | Typically ranges from 50 to 500 microns, depending on the application. |
Deposition Rate | Ranges from 0.5 to 3 kg/hour, influenced by the material and laser power. |
Accuracy | High precision with tolerances in the range of ±0.1 mm or better. |
Flexibility | Capable of handling complex geometries and multiple materials in a single build. |
Scalability | Suitable for both small and large components, from prototypes to production. |
The combination of these properties and characteristics makes LMD a powerful tool for manufacturing and repairing high-performance metal components.
Advantages and Limitations of Laser Metal Deposition (LMD)
Like any manufacturing process, LMD has its strengths and weaknesses. Here’s a breakdown of the advantages and limitations to help you determine if LMD is the right fit for your needs.
Advantages:
Advantage | Explanation |
---|---|
Precision and Accuracy | LMD can produce highly detailed components with tight tolerances. |
Material Efficiency | Minimal waste is generated, making it a cost-effective option. |
Versatility | Capable of processing a wide range of materials, including metals that are difficult to machine. |
Repair Capabilities | LMD excels at repairing high-value components, extending their life and reducing downtime. |
Customization | Easily customizable for small production runs or bespoke parts. |
Limitations:
Limitation | Explanation |
---|---|
High Initial Costs | Equipment and setup costs can be significant, making it a considerable investment. |
Surface Finish | Often requires post-processing to achieve a smooth surface. |
Complexity of Operation | Requires skilled operators and precise control of parameters. |
Heat Affected Zones | The laser can introduce heat affected zones that may alter the material properties. |
Material Limitations | Not all materials are suitable for LMD, especially those with low laser absorptivity. |
Understanding these pros and cons will help you make an informed decision when considering LMD for your manufacturing needs.
Process Parameters Influencing Laser Metal Deposition (LMD)
The quality of components produced via Laser Metal Deposition is highly dependent on several process parameters. These parameters must be carefully controlled to ensure the desired mechanical properties, surface finish, and overall performance of the final product.
Key Process Parameters:
Parameter | Description | Influence on Final Product |
---|---|---|
Laser Power | The amount of energy provided by the laser, typically measured in watts. | Higher power increases deposition rate but may cause overheating. |
Scan Speed | The speed at which the laser moves across the substrate. | Faster speeds can reduce heat input but may lead to incomplete fusion. |
Powder Feed Rate | The rate at which metal powder is delivered to the melt pool. | Higher rates increase deposition efficiency but can lead to porosity if not controlled. |
Shielding Gas Flow | The flow rate of inert gas used to protect the molten pool from oxidation. | Adequate shielding prevents oxidation and contamination. |
Laser Spot Size | The diameter of the laser beam on the substrate. | A smaller spot size improves precision but can slow the process. |
Overlap Ratio | The degree of overlap between adjacent laser passes. | Higher overlap ensures uniformity but can increase processing time. |
Substrate Preheating | The temperature of the substrate before deposition begins. | Preheating reduces residual stresses and cracking. |
Each of these parameters plays a critical role in determining the quality and consistency of the LMD process. For instance, improper laser power or scan speed can result in defects such as porosity, cracking, or poor adhesion between layers.
Optimizing LMD Parameters for Best Results
To achieve the best results with LMD, it’s crucial to optimize these parameters for each specific application and material. This often involves a combination of experimentation and simulation to find the sweet spot where the process is both efficient and produces high-quality parts.
For example, when working with a high-strength alloy like Inconel 625, controlling the laser power and scan speed is essential to avoid overheating, which can lead to undesirable microstructural changes. Conversely, when using a more forgiving material like stainless steel 316L, the focus might be more on optimizing the powder feed rate and shielding gas flow to maximize deposition efficiency.
Advanced Materials Used in Laser Metal Deposition
Beyond the common metal powders listed earlier, LMD can also work with more specialized materials. These advanced materials are used in applications where standard metals might not meet the necessary performance criteria, such as extreme temperatures, corrosive environments, or specific mechanical requirements.
Additional Advanced Metal Powders for LMD:
Metal Powder | Composition | Properties | Characteristics |
---|---|---|---|
Nickel Alloy 263 | Nickel-chromium-cobalt-molybdenum alloy | High-temperature strength, oxidation resistance | Ideal for turbine blades, combustion liners |
Haynes 282 | Nickel-iron-chromium-molybdenum-titanium alloy | High creep resistance, excellent weldability | Used in aerospace, power generation |
Tantalum (Ta) | Pure tantalum | Excellent corrosion resistance, biocompatibility | Suitable for chemical processing, medical devices |
Niobium (Nb) | Pure niobium | High melting point, good superconducting properties | Used in superconductors, aerospace components |
Tungsten (W) | Pure tungsten | Extremely high melting point, high density | Ideal for high-temperature applications |
Inconel 738 | Nickel-chromium-cobalt-aluminum alloy | Exceptional oxidation resistance, high strength | Used in hot-section gas turbine components |
Rene 41 | Nickel-chromium-cobalt-aluminum alloy | Superior high-temperature strength, oxidation resistance | Common in aerospace, gas turbines |
Ti-5553 | Titanium-aluminum-molybdenum-vanadium-chromium alloy | High strength, toughness, good corrosion resistance | Used in aerospace, military applications |
Cobalt Alloy Stellite 6 | Cobalt-chromium-tungsten-carbon alloy | Excellent wear resistance, good corrosion resistance | Ideal for valve seats, cutting tools |
Ni-Cr-B-Si (Colmonoy 88) | Nickel-chromium-boron-silicon alloy | Hardness, wear resistance, good corrosion resistance | Used in surface coating, repair applications |
These advanced materials are chosen for their unique properties that make them suitable for highly demanding applications. For example, Tantalum’s excellent corrosion resistance makes it ideal for chemical processing equipment, while Tungsten’s extreme melting point makes it a top choice for components exposed to very high temperatures.
Comparison of Advanced Materials
Comparing these advanced materials reveals how specific alloys can outperform others in certain conditions, making them more suitable for particular applications.
Material | Strength | Temperature Resistance | Corrosion Resistance | Wear Resistance | Application Suitability |
---|---|---|---|---|---|
Nickel Alloy 263 | High | Excellent | Moderate | Good | Turbines, high-temperature components |
Tantalum | Moderate | High | Excellent | Moderate | Chemical processing, medical devices |
Tungsten | Extremely High | Outstanding | Moderate | Excellent | High-temp applications, aerospace |
Cobalt Alloy Stellite 6 | Moderate | Good | Good | Excellent | Cutting tools, valve seats |
Inconel 738 | Very High | Excellent | Good | Moderate | Gas turbine components, aerospace |
These comparisons can help manufacturers choose the right material based on their specific needs, balancing factors like strength, temperature resistance, and cost.
Applications of Advanced Materials in LMD
The use of advanced materials in LMD extends its applicability to highly specialized fields where traditional manufacturing methods or materials would fail. These materials can meet stringent requirements, offering enhanced performance, longevity, and reliability in critical applications.
Specialized Applications:
Industry | Application | Material Used | Advantages |
---|---|---|---|
Aerospace | Combustion chamber liners, turbine blades | Nickel Alloy 263, Haynes 282 | High-temperature resistance, excellent mechanical properties |
Medical | Custom implants, surgical tools | Tantalum, Cobalt-Chrome (Co-Cr) | Biocompatibility, corrosion resistance |
Energy | Nuclear reactor components, wind turbine shafts | Tungsten, Rene 41 | Radiation resistance, strength under stress |
Defense | Armored vehicles, military-grade hardware | Ti-5553, Tungsten | High strength, toughness, weight reduction |
Automotive | High-performance engine components, heat exchangers | Inconel 738, Titanium Ti-6Al-4V | Heat resistance, strength, reduced weight |
Oil & Gas | Downhole tools, valve seats | Cobalt Alloy Stellite 6, Ni-Cr-B-Si | Wear resistance, durability in harsh environments |
Electronics | Semiconductor components, heat sinks | Niobium, Tungsten | Thermal conductivity, high-temperature capability |
Each application benefits from the specific properties of the advanced materials used. For instance, in the aerospace industry, the high-temperature resistance of Nickel Alloy 263 ensures that turbine blades maintain their integrity even under extreme operating conditions.
Standards and Specifications for LMD Materials
When selecting materials for Laser Metal Deposition, adherence to industry standards and specifications is essential to ensure quality, performance, and safety. Different industries have specific requirements that materials must meet, and these standards guide the selection process.
Industry Standards for LMD Materials:
Material | Standard/Specification | Industry | Key Requirements |
---|---|---|---|
Inconel 625 | ASTM B443, AMS 5599 | Aerospace, Oil & Gas | High temperature, corrosion resistance, mechanical properties |
Titanium Ti-6Al-4V | ASTM F136, AMS 4911 | Medical, Aerospace | Biocompatibility, mechanical strength, fatigue resistance |
Stainless Steel 316L | ASTM A240, ISO 5832-1 | Medical, Food Processing | Corrosion resistance, mechanical properties |
Cobalt-Chrome (Co-Cr) | ASTM F75, ISO 5832-4 | Medical | Wear resistance, biocompatibility |
Tungsten (W) | ASTM B777, MIL-T-21014 | Defense, Aerospace | High density, high-temperature resistance |
Aluminum AlSi10Mg | ASTM B209, EN 485 | Automotive, Aerospace | Lightweight, good thermal conductivity |
Nickel Alloy 263 | AMS 5872, ASTM B637 | Aerospace | Oxidation resistance, high strength at elevated temperatures |
Rene 41 | AMS 5545, ASTM B435 | Aerospace | High-temperature mechanical properties |
Niobium (Nb) | ASTM B392, AMS 7850 | Electronics, Aerospace | Superconductivity, thermal stability |
Tantalum (Ta) | ASTM B708, AMS 7831 | Medical, Chemical Processing | Corrosion resistance, biocompatibility |
These standards ensure that the materials used in LMD are of consistent quality and meet the necessary performance criteria for their intended applications. For example, ASTM F136 ensures that Titanium Ti-6Al-4V used in medical implants is safe and effective for long-term use in the human body.
Choosing the Right Metal Powder for Laser Metal Deposition
Selecting the right metal powder is a critical step in the LMD process. The choice of powder directly affects the quality, performance, and cost of the final product. Factors such as material properties, application requirements, and cost considerations all play a role in this decision-making process.
Factors to Consider:
Factor | Description | Impact on Selection |
---|---|---|
Application Requirements | The specific needs of the final product, including mechanical properties, environmental conditions, and lifespan. | Dictates material selection based on performance criteria. |
Material Properties | Properties like melting point, thermal conductivity, and strength. | Determines process parameters and final part quality. |
Powder Morphology | The shape and size distribution of the powder particles. | Affects flowability, packing density, and layer uniformity. |
Cost Considerations | The cost of the metal powder relative to the project’s budget. | Balances material performance with economic feasibility. |
Supplier Availability | The availability of the metal powder from reliable suppliers. | Ensures consistent supply and quality for production. |
Standards Compliance | Adherence to industry standards and specifications. | Ensures quality and safety of the final product. |
Making an informed decision about the metal powder requires a balance between technical requirements and cost. For instance, while Tungsten offers superior temperature resistance, it is also more expensive than other options like Stainless Steel 316L, making it more suitable for high-end applications where performance justifies the cost.
Suppliers and Pricing for LMD Metal Powders
Sourcing the right metal powder from a reliable supplier is crucial for successful LMD. Suppliers offer a wide range of powders with varying prices depending on factors like material composition, purity, and particle size distribution.
Leading Suppliers and Pricing:
Supplier | Metal Powder | Price Range (per kg) | Special Features |
---|---|---|---|
Carpenter Technology | Nickel Alloy 263, Haynes 282 | $300 – $500 | High-quality aerospace-grade powders, consistent particle size. |
Höganäs AB | Stainless Steel 316L, Inconel 625 | $50 – $200 | Wide range of alloys, excellent flowability. |
LPW Technology | Titanium Ti-6Al-4V, Aluminum AlSi10Mg | $250 – $450 | Custom powders, strict quality control for additive manufacturing. |
Oerlikon Metco | Cobalt Alloy Stellite 6, Rene 41 | $400 – $600 | High-performance powders, optimized for wear resistance. |
AP&C (GE Additive) | Ti-5553, Tantalum | $500 – $800 | Aerospace and medical grade powders, biocompatibility. |
Sandvik | Inconel 738, Tungsten | $200 – $700 | High-temperature alloys, extensive testing and certification. |
Praxis Powder Technology | Niobium, Cobalt-Chrome (Co-Cr) | $300 – $600 | Specialized powders for medical and electronic applications. |
GKN Additive | Nickel Alloy 263, Haynes 282 | $300 – $500 | Customized powder blends, excellent oxidation resistance. |
Tekna | Aluminum AlSi10Mg, Stainless Steel 316L | $50 – $150 | Spherical powders, optimized for additive manufacturing. |
VIGA | Tantalum, Tungsten | $500 – $900 | High-purity powders, tailored for specific applications. |
Prices vary widely depending on the material and supplier, reflecting differences in purity, production method, and market demand. For example, Tantalum and Tungsten powders are on the higher end of the price spectrum due to their complex processing and high demand in specialized industries.
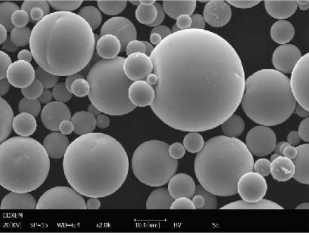
Comparing Laser Metal Deposition to Other Additive Manufacturing Techniques
Laser Metal Deposition is just one of many additive manufacturing techniques available today. Understanding how LMD compares to other methods can help you choose the best process for your specific needs.
Comparison with Other Additive Manufacturing Techniques:
Technique | Material Flexibility | Surface Finish | Speed | Precision | Cost | Applications |
---|---|---|---|---|---|---|
Laser Metal Deposition | High | Moderate | Medium | High | High | Aerospace, repair, complex geometries |
Selective Laser Sintering (SLS) | High | Moderate | Medium | High | Moderate | Prototyping, small batch production |
Electron Beam Melting (EBM) | Medium | Moderate | Medium | High | High | Aerospace, medical implants |
Fused Deposition Modeling (FDM) | Low | Low | High | Low | Low | Prototyping, consumer products |
Direct Metal Laser Sintering (DMLS) | High | High | Medium | Very High | High | Medical, aerospace, intricate parts |
Binder Jetting | Medium | Low | High | Medium | Moderate | Large parts, casting molds |
Directed Energy Deposition (DED) | High | Moderate | Medium | High | High | Repair, large components, aerospace |
LMD stands out for its ability to work with a wide range of materials and its precision in creating complex geometries. However, it tends to be more expensive and slower compared to other techniques like FDM, which is more suited for rapid prototyping with less demanding material requirements.